Theme 1: 2019-2021 work
Following on from part 1 of our Theme 1 summary, see some of the work our modellers got up to from mid-2019 to mid-2021, including the papers we published during this period.
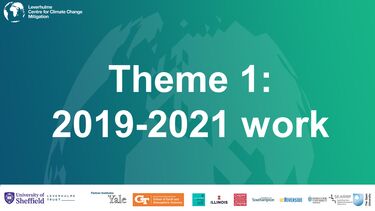
Don’t forget to catch up on part 1 from 2016 to 2019 here, and then read part 3, which follows mid-2021 to mid-2023 work, here.
Jump to:
Towards assessing the role of ERW in achieving the UK’s net zero emissions by 2050
Geochemical modelling of soil-plant weathering processes
Modelling atmospheric chemistry-climate feedbacks during CO2 draw-down with enhanced weathering
Impacts of climate sensitivity to warming and carbon dioxide removal signals
Unlocking the Future of Enhanced Rock Weathering Modelling
2019-2020
Towards assessing the role of ERW in achieving the UK’s net zero emissions by 2050
Over the past year, Dr Euripides Kantzas, Dr Peter Wade and Dr Mark Lomas have refined our 1-D vertical reactive transport with steady-state flow dynamics and a source term representing rock grain dissolution. The model enables us to simulate carbon dioxide removal through enhanced rock weathering on a national scale. It played a crucial role in our recent collaborative research published in Nature.
In 2020 their focus shifted to the United Kingdom. To this end, they’ve focused on agriculture, a sector notoriously challenging to decarbonise, contributing substantially to national N2O and methane emissions – and how ERW deployed on agricultural lands can contribute to the UK’s GGR removal targets.
The UK investigation necessitates a meticulous approach, with the weathering model operating at high spatial and temporal resolutions. Basalt mineralogy was derived from samples collected from UK basalt quarries by PhD student Amy Lewis in Theme 2. Collaborative efforts extend to the integrated assessment modelling work led by Prof Neil Edwards' team in Theme 4, ensuring a holistic understanding of ERW's potential within the UK context.
Our research delves into the intricate dynamics of nitrogen cycling, influenced by both inorganic and organic fertilisers, and their impact on silicate rock weathering rates in soils. Based on Dr Lyla Taylor's understanding of soil nitrogen transformations and Dr Maria Val Martin's knowledge of how these processes are implemented in the Community Land Model (CLM5), we can now represent their effects on the geochemistry of weathering. By preparing driving datasets and coupling high-resolution spatial UK data with simulations from the latest version of the CESM Earth system model, we aim to capture simulations of ERW deployment under different socio-economic scenarios.
Facilitating these particular modules of the model is ºù«Ӱҵ's newest High-Performance Computer cluster, offering enhanced computational performance ideal for large-scale simulations – the model is ready to be benchmarked.
Geochemical modelling of soil-plant weathering processes
Dr Peter Wade's work involved the construction of a sophisticated 1-D reactive transport model (RTM) simulating the dynamic interactions within a soil column. This model integrates various factors such as monthly variable rates of saturated flow-through, weathering of basaltic and soil minerals, precipitation of solids, and adsorption of cations onto soil components like clays, organic matter, and iron phases. This model was applied in Mike Kelland's mesocosm experiment and utilised to calibrate the weathering code developed by Mark for the global model described above.
Dr Lyla Taylor developed code to optimise this model based on feedback from reviewers of Mike’s paper, ensuring its accuracy and reliability. One enhancement was the automation of the model's execution, optimising the reactive surface area of applied minerals and allowing for the presentation of confidence intervals in the results. Lyla’s involvement in the Illinois Energy Farm team has led to the development of a MATLAB interface, streamlining the generation of geochemical and carbon-balance time-series using a PHREEQC model.
Modelling atmospheric chemistry-climate feedbacks during CO2 draw-down with enhanced weathering
Maria continued to investigate interactions between atmospheric chemistry, climate dynamics, and ERW. One significant development involved the implementation of an asynchronous coupling method, essential for running our Earth System Model CESM. This method incorporated changes in soil pH resulting from long-term basalt application to croplands, as estimated by our enhanced weathering model. By assessing the performance of this new method, we aimed to quantify the impact of decreasing soil emissions of N2O and nitrogen oxides (NOx) via ERW on the formation of surface ozone, associated crop damage, and the radiative forcing of climate.
Zooming in on a regional scale, we enabled the global land model CLM5 to conduct simulations specifically tailored to the UK, at a relatively high horizontal resolution of 21 x 30 km2. Initial evaluations of our regional modelling framework yielded promising results. For instance, our system accurately simulated soil N2O emissions, closely matching estimates reported by BEIS and the Inventory Project. Preliminary calculations suggested that ERW could potentially reduce cropland N2O emissions by approximately 8 million tons of CO2 equivalent per year, representing a significant reduction of about 50% compared to current emissions from farmland. Such reductions not only mitigate climate impacts but also hold the potential to enhance overall agricultural sustainability.
Impacts of climate sensitivity to warming and carbon dioxide removal signals
Researchers Katherine Turner and Prof Ric Williams, alongside the Leverhulme Centre for Materials Science, embarked on a collaborative project focusing on the impacts of climate sensitivity to warming and carbon dioxide removal signals.
Utilising an idealised atmosphere-ocean model equipped with ocean overturning circulation and carbonate chemistry, we explored various climate engineering scenarios, including those akin to carbon capture and storage (CCS) and ERW. This model allowed us to manipulate parameters to uncover the underlying controls governing the climate system's response to positive and negative carbon emissions.
The findings revealed a notable delay in peak warming, influenced by the strength of climate feedbacks and characterised by the climate feedback parameter. A lower climate feedback parameter, as observed in recent high-complexity Earth System models, resulted in a prolonged delay between peak cumulative carbon emissions and peak warming, spanning from decades to over a century. This delay hinges on how ocean circulation affects continued heat absorption once emissions cease.
Katherine and Ric discovered that the impact of climate engineering interventions, such as CCS or ERW, also experiences a delay after their cessation, with this delay extending beyond that of peak warming. Although independent of the climate feedback parameter, the timing of this delay varies across different emissions scenarios and ocean overturning strengths.
These findings underscore the critical importance of understanding the climate system's heat uptake behaviour in decision-making processes surrounding the deployment of climate engineering strategies.
Theme 1 papers we published in the period July 2019 to June 2020 include:
- Lawrence, D. M. et al (including Val Martin, M). (2019) . Journal of Advances in Modeling Earth Systems, 11, 4245-4287.
- Beerling, D. J. (2019) Plants, People, Planet. 1:310-314. Published 28 August 2019.
- Andrews, M. G., and Taylor, L. L. (2019) . Elements, 15, 253-258. Published 1 August 2019.
- MacDougall, A. H., Frölicher, T. L., Jones, C. D., Rogelj, J., Matthews, H. D., Zickfeld, K., Arora, V. K., Barrett, N. J., Brovkin, V., Burger, F. A., Eby, M., Eliseev, A. V., Hajima, T., Holden, P. B., Jeltsch-Thömmes, A., Koven, C., Mengis, N., Menviel, L., Michou, M., Mokhov, I. I., Oka, A., Schwinger, J., Séférian, R., Shaffer, G., Sokolov, A., Tachiiri, K., Tjiputra, J., Wiltshire, A., and Ziehn, T. (2020) . Biogeosciences, 17, 2987–3016. Published 15 June 2020.
- Kelland, M. E., Wade, P. W., Lewis, A. L., Taylor, L. L., Sarkar, B., Andrews, M. G., Lomas, M. R., Cotton, T. E. A., Kemp, S. J., James, R. H., Pearce, C. R., Hartley, S. E., Hodson, M. E., Leake, J. R., Banwart, S. A., and Beerling, D. J. (2020) Global Change Biology, 26, 3658–3676.. Published 21 April 2020.
2020-2021
Unlocking the Future of Enhanced Rock Weathering Modelling
Recent advances in our modelling have revolutionised our understanding of how basalt rock powder applications impact agricultural and forested regions. Building upon previous models, which primarily focused on factors like soil temperature and particle size, our advances this year represent a significant leap forward in our ability to simulate and predict the complex interactions between minerals, soil, and vegetation.
Key advances in our ERW model, as highlighted by Beerling et al. (2020) and Kantzas et al., include:
- Plant and fungi influence: New empirical rate laws now quantify how plants and their mycorrhizal fungi associations directly affect silicate mineral surfaces, accelerating their dissolution and the production of alkalinity.
- Particle size distribution: Understanding the impact of mechanical grinding on basalt rock particle size distribution and its subsequent effect on dissolution rates.
- Soil solution chemistry: Examining how soil solutions nearing mineral solubility limits can either inhibit mineral dissolution or initiate the precipitation of secondary minerals like iron and aluminium hydroxides.
- Soil acidification: Considering the ramifications of soil acidification due to fertiliser addition on soil pH, mineral dissolution, and the carbon-nitrogen cycle.
- Phosphorus/Potassium co-release: Recognising the co-release of mineral phosphorus and potassium from basalt, which serves as a crucial nutrient for crop production.
Advances in our computational methods, as described by Beerling et al. (2020), have enabled more comprehensive assessments of ERW's impact on agricultural land. By reducing computational burdens and calibrating simplified empirical functions, we can now simulate mineral weathering kinetics and inhibition more efficiently.
Additional model developments involved the completion of a specialised geochemical reactive transport model tailored to simulate basalt weathering in bench-scale laboratory column reactors. This model serves as a powerful tool, allowing us to interpret detailed experimental data sets and quantify CO2 removal within controlled laboratory environments (Kelland et al., 2020). Building on this work, our PhD student Amy Lewis modelled the dissolution of six basaltic rocks with differing mineralogy, providing insights into the extent and timescale of CO2 removal in soil environments (Lewis et al. 2021). By assessing dissolution rates and CO2 removal potential across a spectrum of basalt rock types available globally, we gain a comprehensive understanding of ERW's applicability.
In tandem, we’ve been developing some time-series, data-driven process models at field scale. For instance, we've integrated streamwater chemistry data with soil greenhouse gas emissions measurements to analyse the long-term greenhouse gas balance associated with silicate mineral treatments applied to forested catchments (Taylor et al., 2021).
This methodology directly informs the development of a novel geochemical crop-soil process model tailored specifically for our Energy Farm site during the LC3M project's fifth year. By leveraging comprehensive datasets from the Energy Farm, we can calibrate and gain a detailed mechanistic understanding of basalt treatment effects on net carbon capture. With appropriate parameterisation, this model holds promise for adaptation to other LC3M field sites.
This year’s advancements underscore the unparalleled sophistication of LC3M ERW modelling methods in the realm of soil weathering processes. Armed with these advanced tools, we can conduct detailed calculations of CO2 removal, unravelling the complexities of plant-soil-basalt-water-atmosphere interactions across diverse landscapes, from individual soil profiles to the expansive reaches of terrestrial planetary surfaces.
Cross-theme work
So far, the advancements in modelling have been linked with Theme 2 to better understand the data collected from laboratory studies on ERW (Kelland et al., 2020). This means that the improvements in modelling help us make sense of the information gathered during experiments conducted in labs to study ERW.
Additionally, these modelling advancements have been integrated with Theme 4. In both the global and UK studies on ERW potential, we've used the scenarios and conditions proposed by Theme 4 regarding the development pathways and public acceptance of ERW. Essentially, this means that we're using the insights and predictions from Theme 4 to guide our research on ERW.
Moving forward, our objectives include assessing the regional and global impacts of ERW on climate change mitigation. We also aim to explore how ERW affects ocean chemistry, particularly in terms of adding alkalinity to counteract the effects of ocean acidification.
Theme 1 papers we published in the period July 2020 to June 2021 include:
- Beerling, D. J., Kantzas, E., Lomas, M. R., Wade, P., Eufrasio, R. M., Renforth, P., Quirk, J., Sarkar, B., Andrews, G., James, R. H., Pearce, C. R., Khanna, M., Koh, L., Quegan, S., Pidgeon, N. F., Janssens, I., Hansen, J., and Banwart, S. A. (2020) . Nature, 583, 242-248. Published 8 July 2020.
- Taylor, L. L., Driscoll, C. T., Groffman, P. M., Rau, G. H., Blum, J. D., and Beerling, D. J. (2021) . Biogeosciences, 18, 169–188. Published 12 January 2021.
- Lewis, A. L., Sarkar, B., Wade, P., Kemp, S. J., Hodson, M. E., Taylor, L. L., Yeong, K. L., Davies, K., Nelson, P. N., Bird, M. I., Kantola, I. B., Masters, M. D., DeLucia, E., Leake, J. R., Banwart, S. A., and Beerling, D. J. (2021) . Applied Geochemistry, 132. Published 21 June 2021.
-
Read parts 1 and 3 here, and then you’ll have a complete summary of our Theme 1 work from 2016 up to 2023. Watch this space for our 2024 update coming soon.